Shell Game: Finding Answers for Acid Waters
Michael W. Fincham
Mike Congrove checks oysters (top) spawned and raised by Oyster Seed Holdings, LLC at its Gwynn Island hatchery down on Virginia’s Piankatank River. Hatcheries like this are supplying larvae (bottom) and disease-resistant oyster seed to a growing number of oyster farmers in Virginia. After episodes of acidic water led to dieoffs of early-stage larvae, hatchery owners began adapting new monitoring techniques and workarounds to keep turning out new oysters for a growing industry. Photographs: Tom Chillemi (top) and Donald Meritt (bottom).
MIKE CONGROVE WAS CRUISING THROUGH A PRETTY GOOD SEASON for spawning oysters when the water in the Piankatank River began to change. He runs a commercial hatchery down on Gwynn Island where this Virginia river meets the Chesapeake Bay. There he takes in water from the river and the Bay, coaxes males and females to spawn, and sells their offspring to a growing number of oyster farmers and gardeners in Virginia.
About the middle of June 2011, his good season hit a big slowdown. At his hatchery, Oyster Seed Holdings LLC, new oyster larvae began dying in large numbers. Larval production dropped from 100 million larvae a week down to 10 million. "We were spawning the same amount of oysters, fertilizing the same number of eggs," he said, "but we were getting extremely low survival rates."
A slim, bearded, and blonde-haired young man, Congrove has worked in the seafood business long enough to know this kind of sudden slowdown could have multiple causes. In this dieoff, however, one major change drew his attention: he was seeing river water with lower levels of pH, a traditional measure of the balance between acidity and alkalinity. The Piankatank and the Chesapeake — at least for a while — had become more acidic.
The Piankatank incident raised a red flag with Congrove — and with operators at half a dozen other Virginia hatcheries who also were seeing a lot of dying and deformed larvae. Their problems here in the Chesapeake sounded a lot like the dieoffs of oyster larvae that struck two Pacific Coast hatcheries back in 2008 and 2009. Researchers and hatchery experts eventually concluded that upwellings from deep in the Pacific had brought acidic water up into the intake pipes for these hatcheries. One result of the acidic influx was a series of oyster dieoffs at Taylor Shellfish Farms in Washington state and at Whiskey Creek Hatchery in Oregon. A second result was a crisis for the entire West Coast oyster industry.
Those two large hatcheries produced much of the oyster seed used by growers from Canada down to Mexico. Oysters seemed to be dying because acidity levels in the ocean were rising.
The West Coast crisis, according to a number of scientists, was another dramatic sign of a disturbing global change. The world's oceans are becoming more acidic as levels of carbon dioxide (CO2) are rising in the earth's atmosphere. The oceans are a great carbon sink absorbing nearly a third of the CO2 that industries and automobiles send into the atmosphere when they burn fossil fuels like coal and oil. All that extra CO2 alters the chemistry of seawater, eventually lowering the levels of calcium carbonate, a key ingredient for many ocean dwellers that use calcium to build skeletons or shells. Around the globe, the poster child for ocean acidification is the coral reef. In the Chesapeake Bay and other coastal waters, it may be the oyster.
For hatchery operators in the Chesapeake, the West Coast crisis comes at a time when oyster farming in the Bay seems to be entering a growth phase after decades of decline. In Virginia, the sale of farmed oysters has increased nearly tenfold since 2004, and growers in 2010 planted three times more seed oysters than ever before. And in Maryland, the state is hoping to imitate the Virginia boom by opening up more areas of Bay bottom for leasing by would-be oyster farmers.
Is rising acidity also a risk for oyster hatcheries and farms here in the Chesapeake? It's a question with no easy answer. An estuary, of course, is not an ocean. The Chesapeake is shallower and less salty than coastal waters along the Pacific, so hatchery operators and farmers here are not exposed to upwellings of acidic waters from the bottom of the ocean. That combination of shallows and lower salinities, however, actually makes the Bay more vulnerable, at least in theory, to rising acidity and declining pH. "At low salinity, the water is less buffered," says Whitman Miller, a researcher at the Smithsonian Environmental Research Center. "So that means any molecule of CO2 has a much bigger effect on a lower-buffered system."
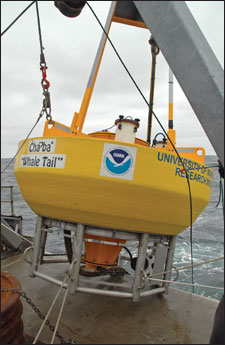
The oyster crash that hit Pacific Coast hatcheries in 2008 drove home the need for close monitoring of coastal and estuarine waters. Federal agencies began adding carbon and pH sensors to open ocean and coastal moorings already in place. International Ocean Observing System (IOOS) partners in the Northwest Association of Networked Ocean Observing Systems (NANOOS) deployed this buoy in 2010 as part of a three-piece observing array to assess issues in the Pacific Northwest, including ocean acidification, hypoxia, harmful algal blooms, and climate change. The coastal buoy aids computer models that predict ocean and atmospheric conditions. Known as “Chá bă, ”the buoy is named for the Native American word (pronounced “chay buh”) for “whale tail.” Data from these buoys can now alert hatchery operators to oncoming acidic upwellings in time to adjust their water intake schedules. Workarounds like this have helped hatcheries increase their production again. Photograph courtesy of John Payne, Pacific Ocean Shelf Tracking Project.
Miller is one of several researchers who've run laboratory studies looking at how rising acidity could affect oysters in the Chesapeake. When he spawned groups of oysters under differing acidities, he did, in fact, find that larvae fared poorly under high acidities. "The larvae grew slower, and they calcified less at high CO2," he says. It was not, he cautions, a real-world test. In the lab he subjected larvae to CO2 levels well above those currently found in the Bay, reaching heights projected for 50 and 100 years from now. And his oyster larvae, he points out, were living under steady-state exposures while larvae in Bay waters would experience fluctuating CO2 levels. Despite these caveats, Miller thinks the risk from rising acidity is real. "The answer is yes," he says. "There is a potential effect."
Acidic water, he found, seems to have its greatest effect on early-stage larvae, a finding that matches the experience of hatchery operators on both the East and West coasts. After males and females spawn, fertilization of new oysters occurs in the water, creating larvae that begin to float and feed. At his hatchery down on the Piankatank River, Mike Congrove has seen new larvae begin to struggle almost immediately as they try to form their first shell and digestive tracts. "It's probably in the first 48 hours or so that these animals are being compromised," he says, "so they don't make it past the first week or so of life."
Those early larval failures in Chesapeake hatcheries are similar to what had happened on the West Coast, according to Alan Barton, the man who was hired to find out what was killing oysters at the Whiskey Creek hatchery in Oregon. "The larvae are dead on day one," he says, "but they don't die until 10 days later."
What Barton discovered has led hatchery operators to change their thinking about the water they let into their tanks. When he went to work at Whiskey Creek, he instinctively focused first on bacteria, specifically a bacterium called Vibrio tubiashi that was known to kill larvae. After engineering a system to filter the bacterium out of the water, he discovered that the larvae kept dying. "We always looked at diseases and bacteria as the culprits killing larvae, and they are," says Barton. "But it turns out those problems get a leg up because of carbon chemistry problems."
The key to the mystery, he decided, was the correlation between dieoffs of larvae and surges of acidic water. When winds blowing from the north push surface waters out to sea, cold water wells up from the deep carrying little or no oxygen, a lot of nutrients, and a lot of dissolved CO2 gases. Not only does this acidic water kill off larvae, it helps support bacteria that also kill larvae. Before these episodes, says Barton, most hatchery operators had not been paying much attention to carbonate chemistry.
Not only are they paying attention now, they are busily looking for workarounds that could keep hatcheries profitable. The first step — monitoring the water coming into the hatchery — seems obvious, but it proved neither easy nor inexpensive. West Coast hatcheries joined together to seek federal grants to install instruments and establish protocols for measuring pH, temperature, salinity, and dissolved oxygen.
Their early monitoring efforts led to their first workaround. CO2 levels, they found, were lowest late in the afternoon, after photosynthesis had spent the day turning CO2 and sunlight and water into plant material and oxygen. Photosynthesis, of course, shuts down during nighttime, but phytoplankton and seagrasses don't turn off their natural respiration. The metabolism that keeps them alive continues around the clock, creating CO2 as waste and releasing it into the water column during all the dark hours. As a result, levels of CO2 are always highest at the start of the day. Hatchery operators now time their water intakes for later in the day.
A second workaround came from weather buoys moored in coastal waters by the U.S. Integrated Ocean Observing System (IOOS), a program led by the National Oceanic and Atmospheric Administration (NOAA). By tracking wind shifts that unleash upwellings, the buoys give early warning alerts to shut down intake pipes. At Taylor's Shellfish Farms, located along inlets off Puget Sound, operators found relief by raising their intake pipes out of deep waters and positioning them near the surface. In 2009, NOAA also began adding carbon and pH sensors to many of its monitoring buoys in coastal waters.
Another solution that helped: adding sodium carbonate and bicarbonate when hatchery waters got too acidic or too alkaline. It's the same ploy the rest of us try when we get an acid stomach: we pop Alka Seltzer to lower the acidity, but its greatest effect is to raise the alkalinity. The chemistry in hatcheries may get a little more complicated than in our stomachs, but the principle seems to be working. "We're probably still in business because of that," says Barton.
Thanks to a combination of research and practical workarounds, West Coast hatcheries have been able to increase their annual production of oyster larvae in recent years.
Those solutions may not be as helpful along the East Coast, where hatcheries in the Chesapeake face a different kind of acid test. The Chesapeake is not the Pacific. Upwellings from the ocean and deposition from the atmosphere are not the major sources of CO2 in the Bay. According to Whitman Miller, "The issue is really how much stuff is coming off the land."
Researcher Whitman Miller on how biology drives CO2 cycles: "In the open ocean, if something dies, it sinks down to the abyss, and that carbon is lost from the surface waters for hundreds of thousands of years. If something dies in the water column in the Chesapeake, it falls a few meters to the bottom, rots, CO2 comes off, and it's back in the water column." Photograph by Michael W. Fincham.
The ongoing overenrichment of the Chesapeake is a well-publicized problem — but it has a little-noted effect. Nutrients running off the land include decaying detritus, animal waste, fertilizer, and sewage, all of which help create blooms of phytoplankton and zooplankton. When these floating plants and animals die, the process of their decomposition lowers oxygen levels, creating well-documented dead zones in the Bay every summer. The surprise kicker: all that decay also recycles CO2 back into the water. And, in the shallows of rivers and creeks, all that CO2 could slow shell growth for young oysters.
Estuarine acidification, it turns out, is quite different from ocean acidification, according to Miller. With acidification in the ocean, the chemistry is driving the biology, but it's the exact opposite with acidification in estuaries. "If you come into the coastal systems," he says, "it is the biology that is driving the chemistry."
Miller saw dramatic evidence of how biology drives CO2 chemistry when he grew test oysters in the Rhode River. He placed one batch next to a dock and another batch next to a nearby salt marsh. Most salt marshes are traps that become biological decay zones for plant material washing off the land, and Miller discovered that CO2 levels coming out of the salt marsh biology were often 10 times higher than levels at the dock. His test oysters grew well near the dock — but not near the marsh. "The ones near the marsh are sort of devastated," says Miller. "Lots of them die. They barely grow at all, even after months and months and months."
There's obviously a lesson there for Chesapeake oyster farming and restoration. "If I were going to site an oyster restoration," says Miller, "it would not be right at a [marsh] location." Oyster growers are already advised not to plant in river areas with high fecal counts. Now they need to avoid low pH levels before planting seed oysters, paying close attention to fringe habitats like sewage outfalls and tidal marshes. Some places in the Bay are better to grow oysters than others.
Some lessons from the West Coast crisis are helpful, some are not. On the Piankatank River, Congrove is already shutting down his intakes when CO2 levels are high and adding sodium carbonate to his hatchery water when pH levels are low. A West Coast solution like early warning buoys is less relevant in the enclosed waters of the Bay. What's needed here, according to Miller, are close readings of water chemistry on smaller, regional scales like the rivers, creeks, and coves where hatcheries or farms might be located.
The search for that kind of Bay-area solution is already underway. This year a coalition of six hatcheries in Virginia is beginning an ambitious effort to monitor a suite of water quality conditions that could be at play in their larvae dieoffs. With funding from the Virginia Secretary for Natural Resources and the Coastal Zone Management Program, hatchery operators will be able to work with more sophisticated gear, train their staff, send samples out for laboratory analysis, and follow a consistent testing protocol at each hatchery.
Costs could run up to $30,000 per hatchery, according to Congrove. That's a hefty outlay, he says, for hatcheries that are "capital limited," but the payoff could be survival and expansion of the growing oyster industry in the state.
A skeptic at work. Like most hatchery operators, Kevin McClarren has seen mysterious dieoffs of newly spawned oyster larvae, but he's unconvinced acidity is at fault. As manager of the Choptank Oyster Company, he runs one of the most successful oyster farms in Maryland and the only privately funded oyster hatchery in the state. "I don't know that anybody who's had a bad season could say it's because of acidity," he says. "I don't know." He's also skeptical about predictions of rising acidity. "People are guessing what the pH is going to be in 30 years." Photograph by Michael W. Fincham.
The chemistry of acidic water will not be the only focus for the monitoring. "We're looking at a number of things we've never looked at in the past," says Dave Kuhn, a researcher at Virginia Tech who will be analyzing data collected from all the hatcheries. In addition to carbonate chemistry like pH and alkalinity, the tests will cover parameters that include algae toxins, pesticides, minerals such as silicates, and nutrients such as ammonia, nitrates, nitrites, phosphorus, and total organic carbon. They will also look for vibrio and other disease-causing microorganisms.
Not every operator, at the moment, is convinced that acidic water is the killer at fault in the Chesapeake. "It's something that's on people's radar," says Kevin McClarren, who manages the Choptank Oyster Company in Cambridge, Maryland. "But pH is not something we really look at in oyster culture." His skepticism is rooted in a familiar experience: oyster spawning can be notoriously variable and the causes for larval dieoffs can be maddeningly mysterious. "There are so many things that are going to do in your larvae. But whether it's one problem or another, we can never figure out," says McClarren. "Once the larvae are dead, the larvae are dead. It's a guessing game after that."
A game changer may yet come out of the new monitoring, a finding that could cut down most of the guessing about dieoffs of oyster larvae. Perhaps some clear correlations will leap out of all that data. Which factors correlate with growth? Which with dieoffs? Mike Congrove has also noticed high ammonia levels in his hatchery water during larvae dieoffs at his Piankatank River hatchery. If larvae are dying at different hatcheries with similar readings — whether it's high levels of acidity or ammonia or some other parameter — then scientists and hatchery operators can start to zero in on the oyster killers.
And they can try more precise solutions, whether it's better filtration to keep out disease vibrios or some new kind of oyster Alka Seltzer to rebalance the acidity of their hatchery waters. "The real purpose of all this monitoring is the practical application," says Congrove. "How can we change the parameters that seem to be problematic?"
Perhaps that's the silver lining in the West Coast crisis. Now that hatchery operators on both coasts have started paying close attention to acidification, carbonate chemistry, and dozens of other factors, they may start finding answers to some old mysteries about what was killing off their baby oysters. And they may find some workarounds to keep their oysters alive and their industry growing.
|